Familiar throughout our international culture, the “Big Bang” is well-known as the theory that scientists use to describe and explain the history of the universe. But the theory is not a single conceptual unit, and there are parts that are more reliable than others.
It’s important to understand that the theory — a set of equations describing how the universe (more precisely, the observable patch of our universe, which may be a tiny fraction of the universe) changes over time, and leading to sometimes precise predictions for what should, if the theory is right, be observed by humans in the sky — actually consists of different periods, some of which are far more speculative than others. In the more speculative early periods, we must use equations in which we have limited confidence at best; moreover, data relevant to these periods, from observations of the cosmos and from particle physics experiments, is slim to none. In more recent periods, our confidence is very, very strong.
In my “History of the Universe” article [see also my related articles on cosmic inflation, on the Hot Big Bang, and on the pre-inflation period; also a comment that the Big Bang is an expansion, not an explosion!], the following figure appears, though without the colored zones, which I’ve added for this post. The colored zones emphasize what we know, what we suspect, and what we don’t know at all.

History of the Universe, taken from my article with the same title, with added color-coded measures of how confident we can be in our understanding. In each colored zone, the degree of confidence and the observational/experimental source of that confidence is indicated. Three different possible starting points for the “Big Bang” are noted at the bottom; note that individual scientists may mean different things by the term.
Notice that in the figure, I don’t measure time from the start of the universe. That’s because I don’t know how or when the universe started (and in particular, the notion that it started from a singularity, or worse, an exploding “cosmic egg”, is simply an over-extrapolation to the past and a misunderstanding of what the theory actually says.) Instead I measure time from the start of the Hot Big Bang in the observable patch of the universe. I also don’t even know precisely when the Hot Big Bang started, but the uncertainty on that initial time (relative to other events) is less than one second — so all the times I’ll mention, which are much longer than that, aren’t affected by this uncertainty.
I’ll now take you through the different confidence zones of the Big Bang, from the latest to the earliest, as indicated in the figure above.
The Green Zone: Confidence
A key clue that the observable patch of the universe was once hot and dense, and cooling as it expanded — i.e. that there was a Hot Big Bang period (preceded perhaps by cosmic inflation, but we don’t need to focus on that yet) — is the presence in the universe of the cosmic microwave background [CMB]. From all parts of the sky, an easily detectable sea of electromagnetic radiation in the form of microwaves (photons with energy about 1000 times less than photons of visible light) is streaming across the universe, and onto the Earth. Not only is the CMB a broad clue as to the universe’s past, it is also a key tool for scientists to use in finding even more precise and focused clues. Much of the history of the observable patch is imprinted in the details of the CMB.
A prediction of the Hot Big Bang is that the microwaves are just a sign of past heat, and of nothing else. Specifically, if you measure the microwave photons’ energy, and plot the amount of energy coming from a patch of sky due to photons of a particular frequency, you should see the same plot (a “black-body spectrum”, as it is called) as you would see for photons emitted by a cold piece of ice or rock or metal. [Yes, ice at 2.7 degrees C above absolute zero does glow in microwaves.] The fact that the COBE satellite’s data agrees closely with the prediction of a black-body spectrum (shown in the figure) is overwhelming evidence that there was a Hot Big Bang going on at the time that atoms first formed, when the observable patch of the universe became transparent to light — a time now believed to be about 380,000 years after the Hot Big Bang began.

Amount of energy in CMB photons as a function of the photons’ frequency. Data from the COBE satellite is the red crosses; the green curve is the prediction of a black-body spectrum.
The most powerful evidence that a simple view of the Hot Big Bang is correct even earlier is the success of the theory (i.e. equations) for nucleosynthesis: the formation of atomic nuclei for the lightest atoms during the Hot Big Bang. (Note the atoms themselves formed hundreds of thousands of years later, when the temperature was cool enough for the nuclei to capture electrons.) The equations of the Hot Big Bang include
- Einstein’s version of gravity and
- the “quantum field theory” used to describe the known particles and non-gravitational forces.
These equations can be used to calculate how abundant certain light atoms should be, if there was a Hot Big Bang. Specifically, one can compute the ratios of how much hydrogen (normal and heavy ["deuterium"]), helium (normal ["helium-4"] and light ["helium-3"]), and lithium should be present in pristine parts of the observable patch, if the atomic nuclei of those elements were mainly forged during the cooling stages of the Hot Big Bang. If the elements other than hydrogen were only formed in stars, then there should be very little helium and virtually no deuterium in the observable patch of the universe. (Deuterium is actually destroyed in stars.) But in a cooling Hot Big Bang, a lot of helium — about 1/4 of the amount of hydrogen — and a small but measurable amount of deuterium should be produced.
These predictions of the Hot Big Bang depend in great detail on our understanding of particle physics and gravity — of the weak nuclear force, the strong nuclear force, the electromagnetic force, the gravitational force (in Einstein’s version of it) and on the properties of neutrinos, electrons, photons, protons and neutrons. And the numbers work! The amount of helium, relative to ordinary hydrogen, is predicted to be in the 20 – 25% range; deuterium is predicted to be in the range of one part in 10,000 to 100,000, helium-3 a factor of 10 smaller than that, and lithium in the part per 10 billion range. The data agree!
![Data showing the prediction for various element abundances (yellow band), data (colored horizontal bands), and predictions (solid colored curves). The fact that the colored curves, colored horizontal bands and the vertical yellow band all meet -- except lithium, which is slightly off but not too far --- indicates that the Hot Big Bang occurred. That Lithium is a bit low has been studied extensively and might mean that a bit of particle physics is missing from the theory. From http://www.einstein-online.info/spotlights/BBN/ ; Adapted from an image by E. Vangioni, Institut d'Astrophysique de Paris]](http://profmattstrassler.files.wordpress.com/2014/03/bbnnucdata.png?w=331&h=416)
Data showing data (colored horizontal bands) and predictions from the Hot Big Bang (solid colored curves) for the abundances of helium-4, deuterium, helium-3 and lithium relative to hydrogen. The quantity on the horizontal axis is essentially the amount of ordinary matter in the observable patch of our universe. The fact that the vertical yellow band meets the colored curves and the corresponding colored horizontal bands — except lithium, where the data is slightly too low — strongly indicates that the Hot Big Bang really occurred. (That Lithium is a bit low has been studied extensively and might mean that a bit of particle physics is missing from the theory; or it might be a problem with the actual measurement of Lithium abundance.) From http://www.einstein-online.info/spotlights/BBN/ ; Adapted from an 2007 image by E. Vangioni, Institut d’Astrophysique de Paris.
That these predictions agree with observations of the distant cosmos (see the figure below) provides a strong argument that the Hot Big Bang really occurred, and was originally hot enough to break apart nuclei. That means that temperatures were initially at least hot enough that the typical particle had an energy of about 0.001 GeV, which would have happened a few minutes after the Hot Big Bang began. More precise measurements even help us understand how much ordinary matter there is in the observable patch of the universe, with results that agree with other measurements of the same quantity.
For this reason, I’ve marked the nucleosynthesis period and what follows it in green, to emphasize that we have strong observational evidence that the Hot Big Bang did occur, and was so hot — hot enough to destroy atomic nuclei — that the atomic nuclei around us today had to form from scratch as the temperature cooled.
The Yellow Zone: Extrapolating a Bit Using Particle Physics
However, for times before nucleosynthesis, the arguments are weaker. We don’t have much direct evidence for what was taking place. But we have a lot of knowledge about particle physics, up to energies of a few hundred GeV, thanks to the Large Hadron Collider [LHC] and its predecessors over the last few decades. So if we assume that the Hot Big Bang started at temperatures so high that a typical particle would have had an energy of a few hundred GeV, we can calculate the observable patch’s properties from that temperature downward. At such a high temperature,
- the Higgs field would have been “off”, so many particles would not have had their usual masses; and
- also, separately, quarks and gluons would have been free to roam.
As the temperature cooled, we can calculate (now that we know the Higgs particle’s mass, and if we assume there aren’t any lightweight particles that we don’t know about) that first the Higgs field would have turned “on”, making the weak nuclear force henceforth weak; and then, a bit later, the strong nuclear force would have become very strong, so that quarks and gluons and anti-quarks would have been trapped henceforth and forever into protons, neutrons, anti-protons and anti-neutrons, as well as other very short-lived hadrons.
However, we can’t yet directly confirm by cosmological observation that this is true. It’s not entirely impossible that there was something odd and unknown about the universe that makes some of these conclusions premature. Someday we may be able to be more certain, but not yet. So in this time period, starting just before the Higgs field turns on and going until nucleosynthesis, we have a highly educated guess as to what was happening, though no direct experimental checks. That’s why I’ve marked this time period in yellow.
The Orange Zone: What Started the Hot Big Bang?
Even earlier? Well, at even higher temperatures we don’t know the particle physics with confidence; we’d need particle accelerators more powerful than the LHC. To figure out what happened in the Big Bang in detail, we have to make assumptions about the particles available, without any way to check them. But still, we are not without information, because we do have an experimental probe: the CMB itself.
It is a remarkable fact that the CMB is incredibly uniform, to one part in 100,000. That’s already information, and explaining why it’s true is one of the arguments in favor of cosmic inflation.
But there’s much, much more information in the imperfections in that uniformity. The non-uniformities, which were discovered by the COBE satellite, have been studied in detail by the WMAP and Planck satellites, along with many other experiments from the ground. And it was in studying the non-uniformities in the CMB and their tiny bit of polarization that BICEP2 made the big (but unconfirmed) discovery that was announced last week (here’s some background information on that discovery, in the form of FAQs, that you may find useful).
The photons in the CMB are astonishingly sensitive. There are very few things that affect them, and fortunately, most of the things that do are relics from the extremely early periods of the observable patch of the universe. It’s an unexpected gift for scientists that this is true! The CMB photons were created about 380,000 years after the Hot Big Bang, and yet they give us insight into the entire period before that, all the way back to a period just before the Hot Big Bang!!! (They also can give us important insights into what happened after 380,000 years, too.) To me, this incredibly sensitivity of the CMB is one of the most amazing natural phenomena in all of science… and it has been the key to progress in cosmology in recent years.
Detailed measurements of the CMB have increasingly given us insight into the zone marked in orange, where we don’t have any particle physics data — just guesses, and calculations based on those guesses. The reason insights have been possible is that our calculations rely on very general properties of the Hot Big Bang and the pre-Hot Big Bang period to make predictions. What I mean by this is that these calculations begin with very simple assumptions about the patterns of non-uniformities that were present at the start of the Hot Big Bang, and then we check if these assumptions give predictions for non-uniformities in the CMB that agree with data. They do! This is shown in the figure below, where predictions of the Hot Big Bang theory, with these simple assumptions about the starting point, are compared with data from the Planck satellite. (Similar but less precise measurements were made before Planck, by WMAP and a number of ground-based experiments.)

Data (red dots) from the Planck satellite, showing the average size of non-uniformities on different angular scales on the sky. The solid curve is the prediction of the current standard cosmological model, which assumes a very simple type of non-uniformity, of the sort that cosmic inflation would produce. The agreement is remarkable, and strongly supports the notion of a Hot Big Bang, but by itself does not confirm there was a period of inflation before the Hot Big Bang.
Thus, simple assumptions about the non-uniformities at the start of the Hot Big Bang seem to be right, more or less. This lends support to the hypothesis of cosmic inflation, which can produce simple non-uniformities. But it’s not convincing, because one can imagine simple non-uniformities arising in some other way. There is at least one alternative — the ekpyrotic universe — that is also largely consistent with this data, and there might have been others that no one has thought of. Meanwhile, there were many qualitatively different forms that cosmic inflation might take, some of which could have been consistent with universes quite different from those with a simple Big Bang. For example, the data has been consistent with universes that, at temperatures a bit higher than reached in the yellow zone in the figure, have more spatial dimensions than the three we’re used to. So the number of possible options in the orange zone has been very large, and the degree of speculation involved has been very high.
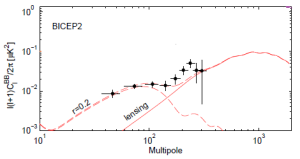
Data from BICEP2, black dots, is compared with the prediction from a model of cosmic inflation (upper dashed line). The prediction is the sum of the solid line (an effect from gravitational lensing in the last few billion years) and the lower dashed line (the effect of gravitational waves from inflation, if inflation involved a very large amount of dark energy.) Had inflation not occurred, or had the amount of dark energy during inflation been small, the dots would have followed the solid “lensing” curve instead of the upper dashed line. See this post for more comments.
This may have changed with BICEP2s new measurement, which gives powerful new evidence for cosmic inflation through inflation’s generation of gravitational waves… assuming the measurement itself, and the interpretation of the measurement, both hold up over time. Since a measurable amount of gravitational waves can only be produced, as far as we know, if a period of inflation occurred and was driven by a very large amount of “dark `energy’ “, BICEP2′s result may rule out all known alternatives to inflation, and significantly narrow down the options for how inflation may have occurred, excluding many of the more radical options as well as many simple ones. As such, it may increase confidence in the inflation hypothesis to change the color of the orange zone to yellow, or even, as more measurements come in and become more precise, to green.
However, let’s not jump to conclusions until the measurement is confirmed with more data and by other observing teams.
The Red Zone: Scientific Guesswork
But BICEP2 can really only tell us about the late stage and exit from inflation. If we go deep into the inflationary period and try to go earlier, into the red zone in the figure, we face two problems:
- we don’t have any experimental probes of this early time, and
- we don’t know which particle physics and/or gravity equations we should be using.
We do not yet have any cosmological observations that access this time period; the CMB photons bring us back to the late stage of inflation, but not further. We cannot infer much yet from CMB measurements about the “inflaton” field (and its particle) that is supposed to play the dominant particle-physics role during inflation, and we have no idea what other types of fields it may interact with, so it is hard to be sure what type of process got inflation started. Meanwhile, theoretical physics doesn’t help much either. We can try assuming that string theory is the true theory of quantum gravity in our world, but if we do that, we still find the equations are hard to solve and give, at best, a variety of possibilities. And trying to work in greater generality, without assuming string theory, doesn’t help; the calculations are still ambiguous and difficult. This doesn’t stop theoretical physicists from making educated, scientific guesses — i.e., speculations backed with concrete equations and calculations. But until we get some data that allows us to distinguish the many possibilities, we can’t say for sure what was actually happening back then.
So if people tells you that the universe started in such and such a way, perhaps “with a singularity” or “with a quantum fluctuation out of nothing” or “in the Big Crunch (i.e. the collapse) of a previous phase of the universe”, remember that they’re telling you about the red zone. They’re neglecting to tell you that what they’re saying is pure theory, with neither an experiment to back it up nor a clear theoretical reason to believe their suggestion is unique and preferable over someone else’s alternative. Only a bit later in cosmic history, once we focus on the late stages of inflation, and forward in time from there to nucleosynthesis, do we have both data (cosmological observation and particle physics collisions) and reasonably reliable theory (Einstein’s theory of gravity plus the Standard Model of particle physics). Our confidence grows as time moves forward, the observable patch of the universe cools, and physics becomes of a sort that we’ve tested in numerous experiments already.
From this, I hope that you can see that the Big Bang Theory really isn’t a single, undifferentiated structure. The most reliable part of the theory is that there was, at one point, a Hot stage of the Big Bang. (Some people call that the Big Bang, in fact.) If BICEP2′s measurement is accurate, and correctly interpreted, then the reliable portion of the theory may then include a period of inflation that preceded the Hot Big Bang. (Some people would call “inflation plus the Hot Big Bang” the “Big Bang”.) But anything before inflation is not in the least reliable… in particular, the notion that the universe’s heat and density increased to the extent that Einstein’s equations have a singularity, presumably indicating that they’re not sufficient to describe what was going on, is an assumption. So that part of the Big Bang Theory may not survive the test of time. You should be prepared, as scientists are, to let it go. After all, from 1960-1980 people had a vision of the early Big Bang that didn’t include something like inflation; their version was significantly adjusted as knowledge was gained, so why shouldn’t ours be?
Moreover, it is quite possible that the start of the universe, which people often refer to as the ultimate Big Bang, may not have had anything “Bang-ish” or even Big about it. Yes, we might even need to let go someday of the idea that the universe truly began with a Big Bang. The scientific community won’t have any problem with this, because many of us have never taken that idea very seriously in the first place. But what about the rest of our society, which seems to hold to this name and notion far harder than do scientists themselves, to the point of (egad!) naming a television show after it?! The loss may be more shocking than necessary…
Filed under: Astronomy, Dark Matter, Higgs, Particle Physics, Quantum Field Theory, Quantum Gravity, Science and Modern Society, String Theory Tagged: astronomy, BICEP2, CMB, cosmology, DarkMatter, fields, gravity, Higgs, inflation, QuantumGravity, quarks, relativity, StringTheory
